Lab-Grown Human Neurons Transplanted into the Rat Brain Grew, Connected, and Promise to Shed Light on Psychiatric Illness
Lab-Grown Human Neurons Transplanted into the Rat Brain Grew, Connected, and Promise to Shed Light on Psychiatric Illness
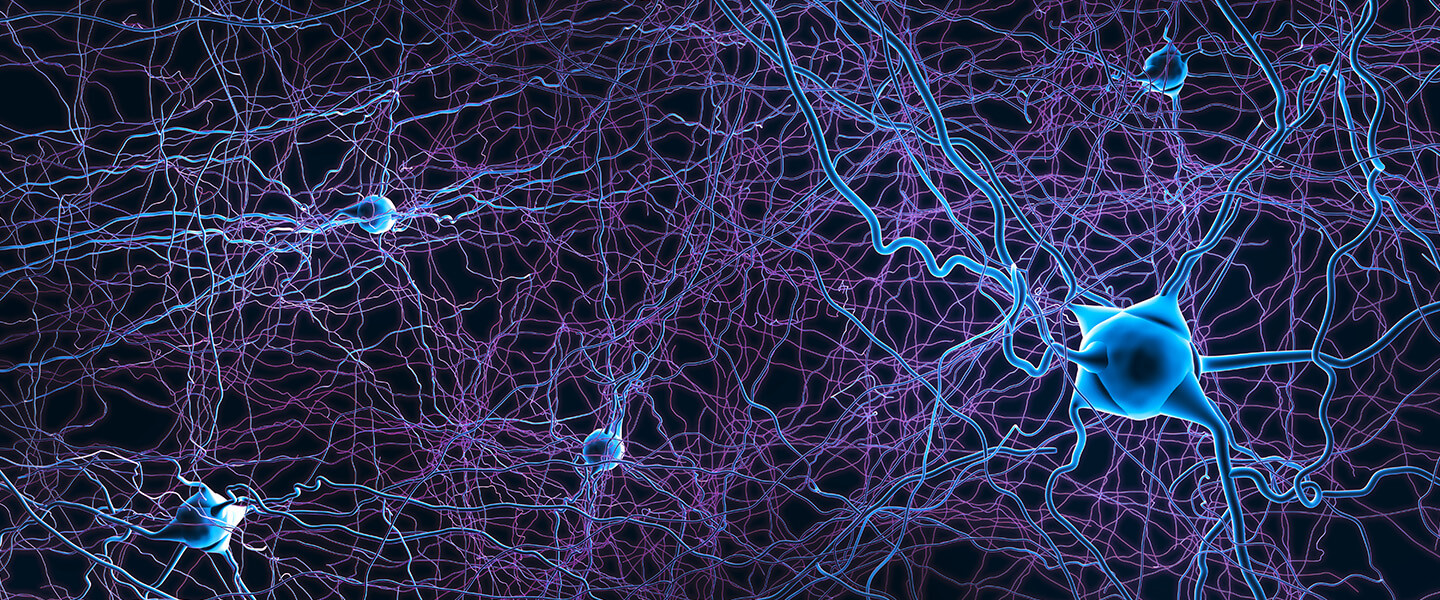
During the last decade, neuroscientists have devised technologies that enable them to grow human brain cells under controlled conditions in the laboratory. A number of BBRF grantees and Scientific Council members have pioneered methods of directing human stem cells—the cells that are the “mothers” of all our cells—to specifically develop as neurons and other brain-cell types.
This remarkable ability, seemingly out of the pages of science fiction, has opened up new possibilities for studying the roots of psychiatric (and other) illnesses. It is possible to direct stem cells to mature, for example, as brain cells, which are not normally accessible in ways that the cells of other bodily organs are. Perhaps even more remarkable is the ability of neuroscientists to harmlessly sample skin cells from an individual suffering from a poorly understood illness with genetic roots, such as autism spectrum disorder or schizophrenia, and to re-program that person’s cells back to a stem cell-like state, and then to redevelop them in the lab as brain cells. This enables researchers to watch the cells as they mature, and potentially to observe problems at the cellular level that mark the beginning or early stages of the disease process.
As exciting as these advances have been, they have proven to have limitations. For example, while human neurons grown in a lab dish can form connections with one another, they don’t mature to stages seen in the living human brain. In the developing brain, in particular—the stage when pathologies in illnesses like autism and schizophrenia likely begin—newly born neurons and other brain cell types receive a wide variety of signals that can’t be mimicked in a lab dish. Among other things, neurons and emerging circuitry directly respond to experience: sights, smells, sounds that a newborn detects and tries to make sense of.
For the last 13 years, Sergiu P. Pasca, M.D., of Stanford University, a 2017 BBRF Independent Investigator and 2012 BBRF Young Investigator, has devoted his efforts to developing and improving ways of growing human brain cells in the lab, and to finding ways of using them more effectively in disease research. In the journal Nature, he and colleagues now report that they have taken a major step forward.
Dr. Pasca’s team has succeeded in taking organoids composed of human cortical cells and transplanting them into the cortex of rats in their first week of life. Cortical organoids are clumps of neurons grown in a dish from human stem-cell precursors.
Dr. Pasca’s aim was to see if transplanted organoids composed of excitatory neurons found in the human cortex would survive in the rodent brain, and if they did, whether the neurons within the organoids would mature and integrate with neurons in the rodent brain, as the host animals matured. The answer to both questions was yes.
In fact, the transplanted organoids grew within 8 months to 9 times their pre-transplantation volume, and, as revealed by MRI, came to occupy about one-third of a hemisphere of the rat brain. Not only were the transplanted neurons larger; they also formed more complex branching connections with other brain cells than did neurons grown in organoids that were not transplanted and continued to be raised in the lab.
Amazingly, this occupation of the rat brain by human cells did not appear to impact the host brain’s workings. The rat brain’s contents were displaced but not disabled. As the researchers had hoped, human neurons within the organoids integrated steadily over time with cells in the rat brain, and in ways more complex than when single human neurons have been implanted in the rodent brain. The rodent hosts receiving the organoid transplants, accommodatingly, steadily supplied the human neurons with nutrients and electrical inputs.
Dr. Pasca’s team targeted the organoids for transplantation into the portion of the rat brain that processes sensory information—the somatosensory cortex. The team conducted experiments demonstrating that the human neurons began to respond to inputs the rats were receiving from their whiskers. In other words, the human cells were integrating functionally into the rat brain and could receive sensory stimulation.
The team went a step further. By conditioning the rats to associate a reward with stimulation of their transplanted human neurons, the rats then began to seek the reward when the team stimulated the human neurons within the rodent brains using optogenetics technology. (Optogenetics employs beams of colored light conducted via a threadlike fiber to active specific neurons.)
In what might be their most consequential success, the team studied cells donated by patients with a rare neuropsychiatric illness called Timothy Syndrome. When transplanted into the rat brain, cortical organoids grown from these cells developed and integrated with the host brain in ways that clearly revealed pathologies consistent with Timothy Syndrome (some of whose symptoms overlap with those of autism spectrum disorder).
This suggests the potential of the cortical transplantation technology to enable researchers to explore pathologies in cells and circuits in a range of psychiatric illnesses with suspected origins in the early phases of life—not only autism, but also schizophrenia, epilepsy and intellectual disability, Dr. Pasca says.
The team included Karl Deisseroth, M.D., Ph.D., of Stanford, a BBRF Scientific Council member, 2013 Goldman-Rakic Prize winner, and 2-time grantee, as well as Felicity Gore, Ph.D., a 2019 BBRF Young Investigator, and Neal D. Amin, M.D., Ph.D., a 2021 BBRF Young Investigator. Dr. Gore was a co-first author of the team’s paper, along with Omer Revah, Ph.D., and Kevin W. Kelley, M.D.