Connecting the Dots, From Genes to Brain Biology to Disorders
Connecting the Dots, From Genes to Brain Biology to Disorders
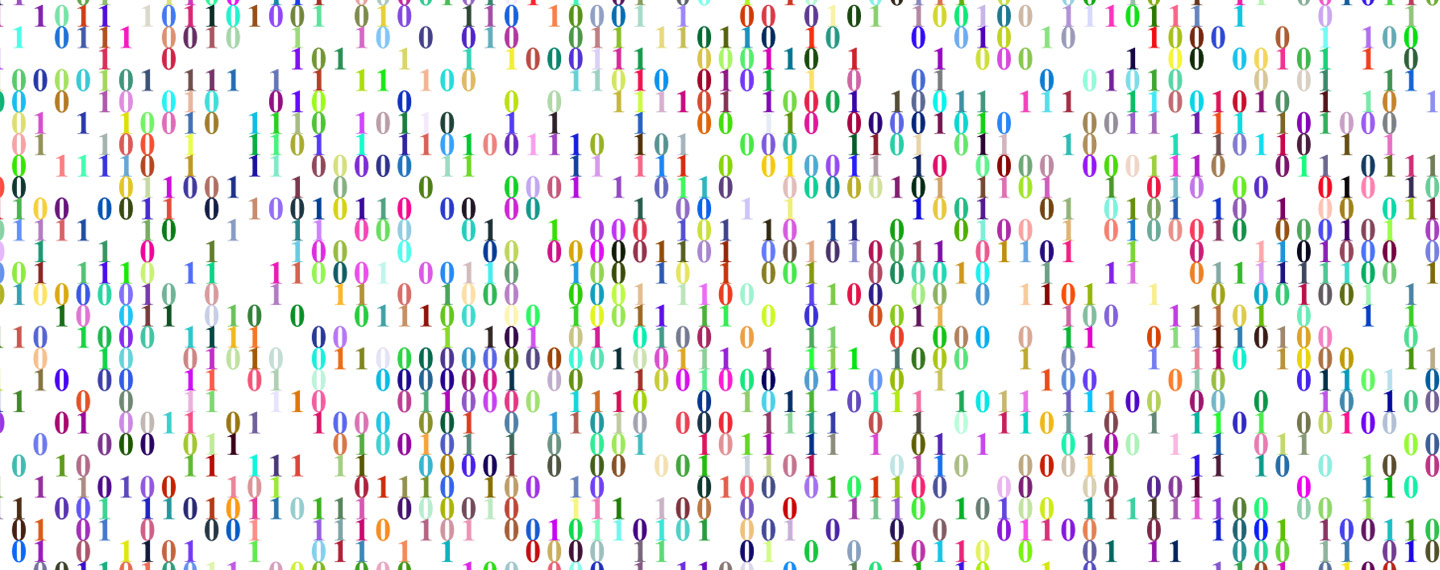
In the first group of 11 papers that PsychENCODE researchers have shared with the scientific community, they demonstrate that by layering different types of data, points of convergence emerge—offering insights about how gene activity in brain cells sheds light on mechanisms that may be involved in causing psychiatric disorders.
This connecting of dots begins to bridge the crucial gap between the discovery of “risk variations” in genome sequencing studies of people with schizophrenia, bipolar disorder, and autism, and the biological processes in the brain that these genetic variations are affecting. These are the kind of insights, it is hoped, that will provide a wealth of new targets for future therapies.
One of the three main papers by the Consortium, which is a good example of its broader approach, made use of 60 postmortem brains unaffected by disease. These brains were probed by many science teams to provide the most comprehensive analysis to date of how gene activity corresponds with the brain’s development. This is valuable in part because these pictures of genetic activity in the developing brain over time can be compared with data gathered from brains of people diagnosed with schizophrenia, autism and bipolar disorder.
THREE LAYERS OF INFORMATION
The team used several kinds of data to make their analysis. One layer of data captured the activity of the full set of human genes, showing how different combinations of genes are important at different moments in life, and most dramatically at the beginning of life, as the brain is just coming into being. This picture of total gene expression is called the transcriptome, a term that refers to the total set of “transcripts” or messages in a cell, each of which is generated when a gene is activated.
The total read-out of gene activity in brain cells was compared at different times—in the early post-conception weeks, at different points in the fetal period, just after birth, and at various subsequent ages, in brains up to 40 years after birth. This comparison formed one layer of the data to be analyzed.
A second layer consisted of what researchers call an epigenetic profile of the brain, again, at different moments in development from the fetal period to adulthood. Epigenetic profiles are a record of how molecules—in this case, methyl groups (CH3)— attach to DNA in the genome, impacting the way genes are expressed. Sometimes, a methyl group sits atop a sequence of DNA in such a way that it prevents a gene from being expressed. Other times, a methyl group attaching to DNA encourages the expression of a gene. Either way, these epigenetic “marks” change the way genes behave, and thus are important to know about in health and illness.
A third layer of data captured what scientists call histone modifications—alterations of proteins that package DNA in the cell nucleus. As with epigenetic marks, these modifications of our genes affect whether specific genes are active or inactive at particular moments in time.
Altogether, these three layers of data gave the PsychENCODE team a picture, in each of the brains they studied, of which genes were active, and the state of biological factors either promoting or preventing gene expression—in different kinds of brain cells, in different parts of the brain, and each in a brain that has been frozen in time at a particular developmental age.
A MAJOR CHANGE IN GENE ACTIVITY, BEFORE BIRTH
What did the layering of these data tell the scientists of PsychENCODE? For one thing, it enabled them to see how gene activity differs in different brain regions over time, in a way that directly reflects brain development.
Very early, in embryonic and early fetal development, gene expression varies greatly both within and across the 16 studied brain regions that are involved in higher-order cognition and behavior.
But this period of great variation is followed by a major transition late in the fetal period and continuing just after birth. In this interval, gene-activity differences diminish both in cells and between brain regions. This fact may be very important. This is the time in brain development when the dentrites along which synapses form begin to branch out It’s also the time glial support cells and astrocytes, which are components of the neural immune system, begin to form.
Why is this important? The researchers note that the time just before this late-fetal transition “coincides with a key developmental period previously associated with the causation of autism spectrum disorder and schizophrenia.” If you want to understand these illnesses, they say, it is almost certainly important to understand how the brain develops and genes express themselves during this crucial period.
RELATING THE ACTIVITY OF “RISK GENES” AND ILLNESSES
The layers of data they collected enabled the PsychENCODE team to be more specific. They describe a group of genes that tend to be activated at the same time in neurons, and that are involved in related biological functions. During the major transition in the brain just before birth, the activity of this set of “co-expressing” genes—a grouping they call ME37— changes more than that of any other grouping of genes in the fetal brain.
The team then noticed that a number of the genes in the ME37 cluster have been identified as “risk genes” in autism, schizophrenia, intellectual disability, and in neurodevelopmental disorders generally.
This suggests how PsychENCODE is taking science beyond the mere identification of “risk genes.” All the layers of information in the study described here, when integrated, converged on the ME37 cluster in particular as being an area of risk for pathology and therefore one that is potentially rich in targets for future therapeutics.
A footnote: the team dedicated their first set of 11 papers “to Pamela Sklar, one of the chief architects and leaders of the PsychENCDOE Consortium, whose ideas resonate throughout our studies.” Dr. Sklar, an M.D., Ph.D., who was Chief of the Division of Psychiatric Genomics at the Icahn School of Medicine at Mount Sinai, died of cancer in 2017. Dr. Sklar was a member of BBRF’s Scientific Council, a 2016 winner of the BBRF’s Colvin Prize, and a 2006 BBRF Independent Investigator and 1998 and 1995 BBRF Young Investigator.
— Written By Peter Tarr
Click here to read the Brain & Behavior Magazine's March 2020 issue